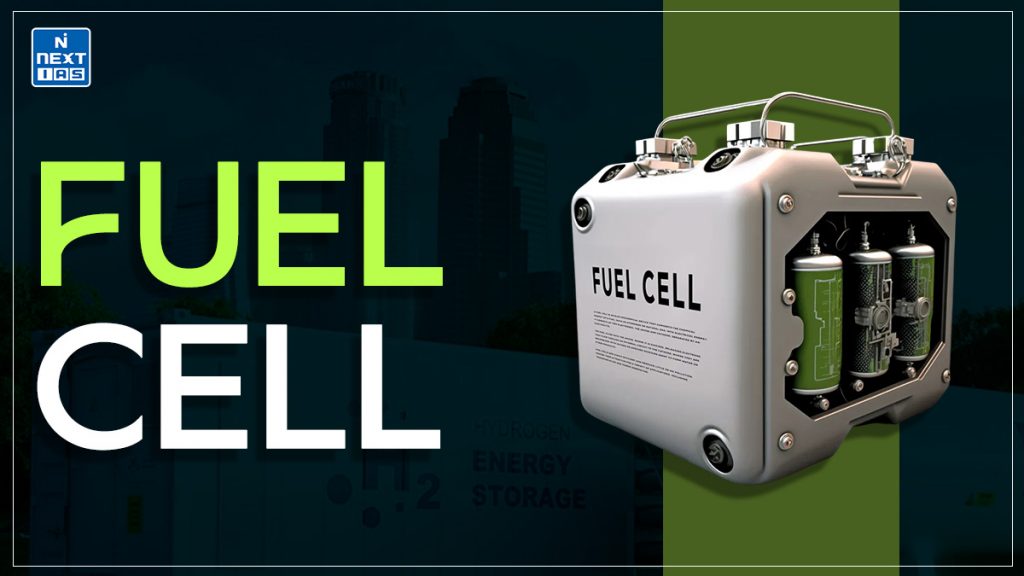
A fuel cell is an electrochemical device that converts chemical energy from fuels, such as hydrogen, directly into electricity through a reaction with oxygen. Unlike traditional combustion engines, fuel cells produce electricity with high efficiency and minimal emissions, making them a promising technology for clean energy and sustainable transportation.
What is a Fuel Cell?
- It is an electrochemical device that converts chemical energy into electrical energy by combining a fuel (usually hydrogen) with an oxidant (typically oxygen from the air).
- Unlike conventional batteries, which store energy, fuel cells produce electricity as long as fuel is supplied. The core reaction involves hydrogen molecules splitting into protons and electrons at the anode, with electrons creating an electric current through an external circuit.
- The protons move through the electrolyte to the cathode, where they combine with oxygen to form water and heat as byproducts. This process is clean, with water being the primary waste, making fuel cells an environmentally friendly alternative to fossil fuels.
- Fuel cells are used in a variety of applications, from powering electric vehicles (like hydrogen-powered cars) to providing electricity in remote locations. They offer advantages like high efficiency, low emissions, and the potential for renewable energy integration.
Types of Fuel Cell
They are devices that convert chemical energy from a fuel into electricity through an electrochemical reaction. Different types of fuel cells are categorized based on their electrolyte, operating temperature, and application. Here are the main types:
Proton Exchange Membrane Fuel Cells (PEMFCs)
- Electrolyte: Proton-conducting polymer membrane
- Operating Temperature: 50°C to 100°C
- Fuel: Hydrogen
- Oxidant: Oxygen or air
- Applications: Transportation (e.g., fuel cell vehicles), portable power, and backup power systems
- Advantages: Compact, lightweight, quick start-up, high efficiency
- Disadvantages: Sensitive to fuel purity and requires expensive catalysts (platinum)
Alkaline Fuel Cells (AFCs)
- Electrolyte: Potassium hydroxide solution (KOH)
- Operating Temperature: 60°C to 90°C
- Fuel: Hydrogen
- Oxidant: Oxygen or air
- Applications: Space applications (e.g., NASA’s Apollo missions), portable power
- Advantages: High efficiency, lower cost catalysts (e.g., non-platinum materials)
- Disadvantages: Carbon dioxide (CO₂) contamination affects performance, limiting outdoor applications
Solid Oxide Fuel Cells (SOFCs)
- Electrolyte: Ceramic material (e.g., yttria-stabilized zirconia)
- Operating Temperature: 500°C to 1000°C
- Fuel: Hydrogen, natural gas, biogas, or other hydrocarbons
- Oxidant: Oxygen or air
- Applications: Stationary power generation, combined heat and power (CHP) systems
- Advantages: High efficiency, fuel flexibility, and cogeneration capability
- Disadvantages: High operating temperature leading to long start-up times and potential material degradation
Phosphoric Acid Fuel Cells (PAFCs)
- Electrolyte: Liquid phosphoric acid
- Operating Temperature: 150°C to 200°C
- Fuel: Hydrogen
- Oxidant: Oxygen or air
- Applications: Stationary power generation, small-scale combined heat and power (CHP) systems
- Advantages: High tolerance to impurities in hydrogen, robust and reliable
- Disadvantages: Moderate efficiency, larger size, and weight
Molten Carbonate Fuel Cells (MCFCs)
- Electrolyte: Molten carbonate salts
- Operating Temperature: 600°C to 700°C
- Fuel: Hydrogen, natural gas, biogas, or other hydrocarbons
- Oxidant: Oxygen or air
- Applications: Large-scale power plants, industrial applications
- Advantages: High efficiency, fuel flexibility, and ability to use CO₂-rich fuels
- Disadvantages: High operating temperature causing material degradation and complex thermal management
Direct Methanol Fuel Cells (DMFCs)
- Electrolyte: Proton-conducting polymer membrane
- Operating Temperature: 50°C to 120°C
- Fuel: Methanol (liquid)
- Oxidant: Oxygen or air
- Applications: Portable electronic devices, small-scale power systems
- Advantages: Easy fuel storage and transportation, suitable for portable use
- Disadvantages: Lower efficiency and performance compared to hydrogen-based PEMFCs
Each type of fuel cell is suited for specific applications based on its operating conditions, fuel source, and efficiency.
Advantages of Fuel Cell
Fuel cells offer numerous advantages due to their efficiency, environmental benefits, and versatility. Below are the key advantages:
- High Efficiency
- It converts chemical energy directly into electrical energy with efficiencies of 40-60%, higher than conventional combustion-based power generation systems.
- When used in combined heat and power (CHP) systems, overall efficiency can exceed 80%.
- Environmental Benefits
- Zero Emissions (with Hydrogen): When hydrogen is used as a fuel, the only byproduct is water, making it an environmentally friendly technology.
- Reduced Greenhouse Gases: Even with hydrocarbons as fuel, fuel cells produce fewer greenhouse gases compared to combustion engines.
- Fuel Flexibility
- Fuel cells can operate on various fuels, including hydrogen, natural gas, methanol, ethanol, biogas, and hydrocarbons.
- This flexibility makes them adaptable for different applications and resources.
- Quiet Operation
- It can operate silently because they have no moving parts in the energy conversion process, making them ideal for residential areas and noise-sensitive environments.
- Scalability
- They are highly scalable and can be designed for a wide range of power outputs, from small portable devices to large power plants.
- Reliable Power Supply
- It provide consistent and reliable power without fluctuations, making them suitable for critical applications like hospitals, data centers, and telecommunications.
- Reduced Dependency on Fossil Fuels
- With hydrogen from renewable sources, fuel cells can decrease reliance on fossil fuels and contribute to energy independence.
- Durability in Harsh Environments
- It operates in extreme temperatures and challenging environments where other power sources might fail.
- Energy Security
- They can function as backup power systems during outages and emergencies, improving resilience in critical infrastructure.
- Potential for Renewable Integration
- Hydrogen for fuel cells can be produced via electrolysis powered by renewable energy, enabling a sustainable energy cycle.
- Compact and Modular Design
- Fuel cells are compact and modular, allowing for easier integration into vehicles, buildings, and portable systems.
While fuel cells offer many advantages, challenges like high costs, fuel production infrastructure, and material limitations need to be addressed for widespread adoption.
Concerns With Fuel Cell
Despite their numerous advantages, fuel cells face several challenges and concerns that hinder their widespread adoption. These concerns include technical, economic, and environmental aspects:
- High Costs
- Materials: Many fuel cells, especially Proton Exchange Membrane Fuel Cells (PEMFCs), rely on expensive materials like platinum as a catalyst.
- Manufacturing: The production process for fuel cells is complex and costly, particularly for high-performance units.
- Fuel Costs: Hydrogen production, storage, and transportation can be expensive, particularly for green hydrogen.
- Hydrogen Infrastructure
- Limited Infrastructure: A lack of widespread hydrogen production, distribution, and refueling infrastructure limits the adoption of hydrogen fuel cells.
- Transportation Challenges: Hydrogen is challenging to store and transport due to its low energy density and the need for high-pressure or cryogenic systems.
- Fuel Production Challenges
- Non-Renewable Sources: Most hydrogen is currently produced from natural gas via steam methane reforming, which emits significant CO₂.
- Energy Intensive: Producing green hydrogen via electrolysis requires substantial energy, often from renewable sources, which are not yet widely available or cost-effective.
- Durability and Longevity
- Degradation: Fuel cell components, such as membranes and catalysts, degrade over time, reducing efficiency and requiring replacement.
- Thermal and Mechanical Stress: High operating temperatures (e.g., in Solid Oxide Fuel Cells) can lead to material degradation and reduced lifespan.
- Efficiency Loss
- While fuel cells are efficient, energy losses can occur during hydrogen production, compression, transport, and conversion, reducing the overall system efficiency.
- Safety Concerns
- Hydrogen Flammability: Hydrogen is highly flammable and poses risks if not handled correctly. Leaks can lead to explosions in confined spaces.
- High Pressure: Storing hydrogen at high pressures (up to 700 bar) introduces mechanical risks.
- Environmental Concerns
- Carbon Footprint of Production: Hydrogen produced from fossil fuels (grey hydrogen) generates significant CO₂ emissions.
- Rare Material Extraction: The extraction and refining of rare materials like platinum and palladium for catalysts have environmental and ethical implications.
- Competition with Other Technologies
- Battery Advancements: Lithium-ion and solid-state batteries are competing technologies for applications like electric vehicles and portable devices, often offering lower costs and easier infrastructure integration.
- Combustion Engines: Conventional engines remain cheaper and more established for many applications.
- Temperature Constraints
- Low-Temperature Fuel Cells: PEMFCs and Alkaline Fuel Cells (AFCs) are sensitive to operating conditions and can freeze in cold climates.
- High-Temperature Fuel Cells: SOFCs and MCFCs require lengthy start-up times and robust thermal management systems.
- Public Awareness and Policy Support
- Lack of awareness about fuel cells and insufficient government policies or incentives hinder investment and adoption.
Addressing these concerns requires advancements in materials, production methods, infrastructure development, and policy frameworks. Collaboration among governments, industries, and research institutions is essential for overcoming these challenges and realizing the potential of fuel cell technology.
Applications of Fuel Cell
Fuel cells have diverse applications across various sectors due to their efficiency, environmental benefits, and adaptability. Here are the primary applications of fuel cells:
Transportation
Fuel cells power various vehicles, offering zero-emission alternatives to traditional internal combustion engines.
- Cars and SUVs: Fuel Cell Electric Vehicles (FCEVs), such as the Toyota Mirai and Hyundai NEXO.
- Buses: Public transit systems in cities around the world are adopting fuel cell buses for cleaner transportation.
- Trucks: Long-haul and delivery trucks are increasingly using hydrogen fuel cells for extended range and quick refueling.
- Trains: Hydrogen-powered trains are being deployed in regions without electrified railways (e.g., Alstom’s Coradia iLint).
- Marine Vessels: Fuel cells are used in boats, ferries, and submarines for quiet and clean operation.
- Aircraft and Drones: Hydrogen fuel cells are being tested for small aircraft and drones to achieve longer flight times with low emissions.
Stationary Power Generation
Fuel cells provide reliable and efficient power for residential, commercial, and industrial use.
- Residential Power: Micro-CHP systems use fuel cells to generate electricity and heat for homes.
- Commercial Power: Fuel cells supply electricity to offices, hotels, and hospitals.
- Industrial Applications: Used in factories and data centers requiring consistent, large-scale power.
- Backup Power Systems: Reliable backup power for telecommunications, data centers, and emergency services.
Portable Power
Fuel cells are used to power portable devices and remote systems where conventional power sources are impractical.
- Consumer Electronics: Fuel cells can power laptops, smartphones, and cameras.
- Remote Applications: Used in off-grid locations, such as weather stations, remote monitoring systems, and military outposts.
- Portable Generators: Ideal for camping, outdoor activities, and disaster recovery operations.
Combined Heat and Power (CHP)
- Fuel cells produce both electricity and heat simultaneously, enhancing overall energy efficiency.
- Applications include residential heating systems, commercial buildings, and industrial facilities.
Industrial Hydrogen Production
It can generate hydrogen for use in industrial processes, such as:
- Chemical production (e.g., ammonia for fertilizers).
- Refining petroleum.
- Metal processing.
Renewable Energy Integration
Fuel cells are used in energy systems that combine renewable energy sources.
- Hydrogen Storage: Excess renewable electricity (e.g., solar, wind) is used to produce hydrogen, which is later converted back into electricity via fuel cells.
- Grid Stabilization: Fuel cells help stabilize power grids by providing clean backup power.
Military Applications
They are used by armed forces for their reliability, quiet operation, and portability.
- Unmanned Vehicles: Power for unmanned aerial, ground, and underwater vehicles.
- Portable Power Units: For field operations and communication devices.
- Submarines: Air-independent propulsion systems for stealthy operations.
Space Exploration
Fuel cells have been used in space missions since the 1960s (e.g., NASA’s Apollo program).
- Spacecraft Power: Fuel cells generate electricity and drinking water for astronauts.
- Space Stations and Rovers: Power systems for long-term space exploration.
Hydrogen Refueling Stations
Fuel cells are employed in some hydrogen stations to generate power on-site, ensuring a sustainable refueling process.
Emerging Applications
Fuel cells continue to find new uses as technology advances:
- Hydrogen-powered agriculture equipment (e.g., tractors).
- Hydrogen trains for non-electrified railway systems.
- Portable medical devices requiring reliable, clean power.
Fuel cells’ versatility and clean energy potential make them increasingly valuable across industries, with continued advancements opening new application areas.
Way Forward
The way forward for fuel cells involves reducing costs through advanced materials and mass production, expanding hydrogen infrastructure, and enhancing renewable hydrogen production. Policy support, research investment, and public-private partnerships are crucial. Promoting awareness, ensuring safety, and integrating fuel cells into energy systems will accelerate adoption for a sustainable, low-carbon future.
Conclusion
Fuel cells are a promising clean energy technology, offering high efficiency, versatility, and environmental benefits. Despite challenges like cost and infrastructure, advancements in materials and hydrogen production are driving adoption. With diverse applications across transportation, power generation, and portable devices, fuel cells play a vital role in the transition to sustainable energy systems.
GS - 3