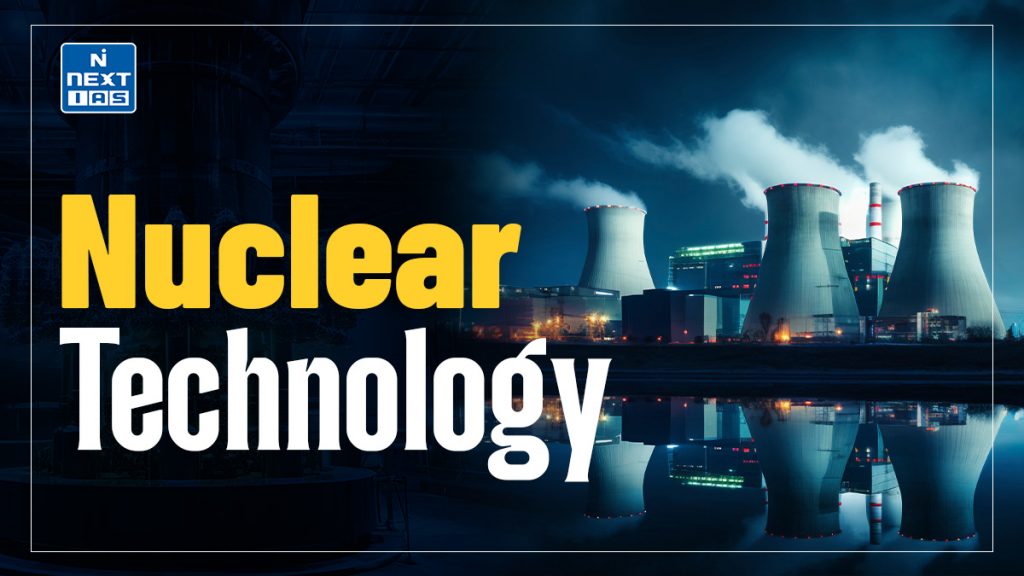
Nuclear technology involves the manipulation of atomic nuclei to release energy for various applications. Its core principles include nuclear fission, where atoms split to produce energy, and nuclear fusion, where atoms combine. This technology powers electricity generation, medical treatments, and scientific research, while also carrying implications for environmental sustainability and safety.
What is Nuclear Technology?
Nuclear technology harnesses the energy released from atomic reactions, primarily through nuclear fission and fusion, to produce energy, conduct medical treatments, and enable scientific advancements.
- Nuclear Fission: This process involves splitting heavy atomic nuclei (like uranium or plutonium) to release substantial energy. It’s the primary reaction in nuclear power plants, which convert this energy into electricity.
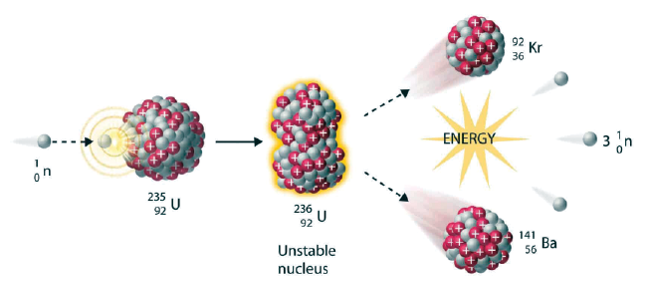
- Nuclear Fusion: Fusion combines light atomic nuclei (like hydrogen isotopes) under high pressure and temperature to form heavier nuclei, releasing even greater energy. While still largely experimental, fusion has the potential to provide a nearly limitless, clean energy source.
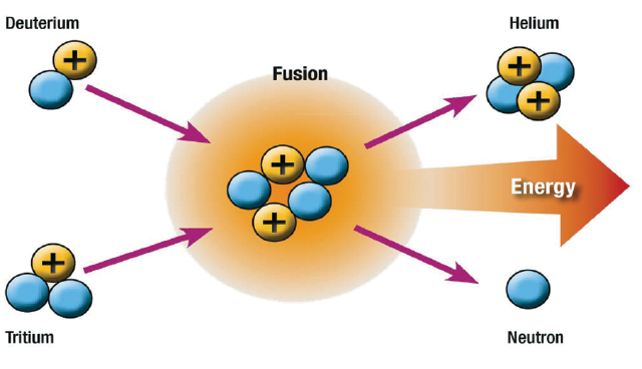
Nuclear Reactors and Its Types
Nuclear reactors are devices used to initiate and control nuclear chain reactions for various purposes, including energy generation, research, and medical applications. They operate by harnessing the energy released from nuclear fission, where heavy atomic nuclei, typically uranium-235 or plutonium-239, split into smaller nuclei upon being struck by neutrons, releasing a significant amount of energy in the form of heat.
There are several types of nuclear reactors, each designed with specific functions and technologies. Below are the primary types:
Pressurized Water Reactor (PWR)
- How it works: In a PWR, water is used as both a coolant and a moderator. The water in the reactor core is kept under high pressure to prevent it from boiling, even though it gets very hot. The heated water is then used to produce steam in a secondary loop, which drives a turbine to generate electricity.
- Use: Common in commercial nuclear power plants around the world.
- Advantages: High safety, mature technology, and widespread use.
Boiling Water Reactor (BWR)
- How it works: Unlike the PWR, the water in a BWR boils directly in the reactor core to produce steam. This steam is used to drive turbines and generate electricity, without the need for a secondary loop.
- Use: Found in several nuclear power plants, especially in the U.S.
- Advantages: Simpler design compared to PWR, as it doesn’t require a heat exchanger.
Heavy Water Reactor (HWR)
- How it works: Uses heavy water (deuterium oxide, D₂O) as a moderator instead of regular water. Heavy water is more effective at slowing neutrons, allowing the reactor to use natural uranium (which is not enriched) as fuel.
- Use: Common in countries like Canada (CANDU reactors) and India.
- Advantages: Can operate with natural uranium, making it ideal for countries with limited access to enriched uranium.
Gas-Cooled Reactor (GCR)
- How it works: Uses carbon dioxide or helium as a coolant and graphite as a moderator. The reactor uses uranium fuel, and the gas coolant is circulated to transfer heat away from the reactor core.
- Use: Historically used in the UK and still used for research reactors.
- Advantages: High thermal efficiency and operates at higher temperatures.
Fast Breeder Reactor (FBR)
- How it works: This type of reactor is designed to breed more fuel than it consumes. It uses fast neutrons to convert fertile materials like uranium-238 into fissile material (like plutonium-239), which can then be used as fuel.
- Use: Primarily for generating electricity and producing more nuclear fuel.
- Advantages: Efficient use of nuclear resources and helps extend the lifespan of uranium.
Molten Salt Reactor (MSR)
- How it works: In an MSR, the coolant and the fuel are both in liquid form. The nuclear fuel is typically dissolved in a molten salt mixture, and the heat generated is transferred directly to a turbine.
- Use: Currently in the experimental or development phase, with research underway in countries like the U.S. and China.
- Advantages: Potential for higher safety, efficiency, and waste reduction.
Small Modular Reactor (SMR)
- How it works: A newer concept, SMRs are smaller in size and output compared to traditional reactors, designed for modular construction and scalability. They are typically designed to be factory-built and transported to sites for easier deployment.
- Use: Still in the development phase, but potential for distributed power generation.
- Advantages: Lower construction costs, flexibility, enhanced safety features.
Liquid Metal-Cooled Reactor (LMR)
- How it works: These reactors use liquid metals like sodium or lead as coolants, which transfer heat more efficiently than water. The liquid metal circulates around the reactor core to carry away the heat generated by fission.
- Use: Research reactors and some experimental power reactors.
- Advantages: Better heat transfer, high operating temperature, and safety.
Supercritical Water Reactor (SCWR)
- How it works: This type of reactor uses supercritical water (water that is heated beyond its critical point) as both a coolant and a moderator. It operates at higher temperatures and pressures, improving thermal efficiency.
- Use: Still under research and development.
- Advantages: Higher efficiency compared to conventional water reactors.
Thorium Reactor
- How it works: Thorium reactors use thorium-232 as fuel, which absorbs neutrons to form uranium-233, which is fissile. This type of reactor can potentially offer safer, more sustainable nuclear energy with fewer long-lived radioactive waste products.
- Use: In research stages but with potential for the future.
- Advantages: More abundant fuel, potentially less waste, and improved safety.
Compact Fusion Reactors
- How it works: A different form of nuclear reactor that aims to replicate the process that powers the sun: nuclear fusion. Unlike fission, which splits atoms, fusion involves combining light atomic nuclei, like hydrogen, to produce energy.
- Use: Still in the experimental stage, with projects like ITER working toward demonstrating the viability of fusion energy.
- Advantages: Cleaner, with little radioactive waste and a potentially limitless energy supply.
Applications of Nuclear Technology
In addition to producing electricity, nuclear technology has a variety of other beneficial applications. They include space exploration, water desalination, agriculture, and medicine.
- Agriculture: To stop dangerous insects from breeding, agricultural workers employ radiation.
- For instance, the Ministry of Food Processing Industries offers subsidies to gamma radiation processing plants that are set up for the gamma radiation processing of food products under the SAMPADA (Scheme for Agro-Marine Processing and Development of Agro-Processing Clusters) scheme.
- Food: Bacteria and other possibly hazardous creatures are eliminated by radiation. The food is not rendered radioactive by this method of sanitation, nor does it significantly alter its nutritional content. The only efficient method for eliminating microorganisms from frozen and uncooked foods is radiation.
- Medical: Inside pictures of the human body are provided by nuclear technologies.
- For example, Nuclear research has enabled doctors to precisely predict the amount of radiation required to kill cancer tumours while causing no harm to healthy cells.
- Space Exploration: Nuclear technology makes deep space exploration possible. Unmanned spacecraft generators use the heat from plutonium to generate electricity and can operate unattended for years. This dependable, long-term source of power propels these spacecraft even as they travel deep into space.
- For example: According to the Nuclear Energy Institute, Voyager 1, which was launched in 1977 to study the outer solar system, is still transmitting data today.
- Water Desalination: Water desalination is the process of removing salt from saltwater in order to make it drinkable. However, this process consumes a lot of energy. Nuclear energy facilities can provide the large amount of energy required by desalination plants to provide fresh drinking water.
Challenges of Nuclear Technology
Nuclear technology offers significant benefits, including clean energy, medical advancements, and industrial applications. However, it also presents complex challenges across various domains:
Nuclear Waste Management
- Long-term Storage: Nuclear waste, especially high-level waste from reactors, remains radioactive for thousands of years. Safe and secure storage solutions are necessary, with the most promising being deep geological repositories. However, finding suitable sites and ensuring they remain secure over centuries is a major challenge.
- Environmental Risks: Leaks or improper disposal can lead to severe environmental contamination, potentially impacting ecosystems and human health.
Nuclear Accidents and Safety
- Disasters: High-profile incidents like Chernobyl, Fukushima, and Three Mile Island show the catastrophic potential of nuclear accidents, which can lead to radiation exposure, contamination, and displacement of communities.
- Safety Protocols: Maintaining robust safety protocols and ensuring reactor safety can be challenging, especially in older reactors or in countries with limited regulatory oversight.
Nuclear Proliferation and Security
- Weapons Development: The same technologies used for peaceful nuclear energy can be diverted to create nuclear weapons. Preventing nuclear proliferation is a key concern, especially with international tensions and countries aiming to develop nuclear arsenals.
- Terrorism and Theft: Securing nuclear materials to prevent theft or sabotage is an ongoing challenge, as nuclear materials can be misused by non-state actors for weapons or dirty bombs.
High Costs and Economic Viability
- Construction Costs: Nuclear plants are extremely costly to build, and construction can take a decade or longer. Additionally, decommissioning old plants is expensive and complex.
- Maintenance and Operational Costs: Nuclear plants require skilled personnel and ongoing maintenance to ensure safety, making them more costly than some renewable sources like wind or solar, which are becoming cheaper.
Environmental and Health Concerns
- Mining and Processing: Uranium mining and processing can harm the environment, producing radioactive waste and contaminating water sources, impacting both ecosystems and local communities.
- Radiation Exposure: Workers in nuclear facilities and communities near nuclear sites face potential radiation exposure, which can increase the risk of cancer and other health issues.
Public Perception and Acceptance
- Fear and Stigma: Nuclear technology often faces public opposition due to its association with radiation, accidents, and weapons. This can lead to political resistance and challenges in obtaining necessary approvals for new projects.
- Misinformation: Misunderstandings about the risks and benefits of nuclear technology often fuel opposition, making it difficult for advocates to promote nuclear energy as a clean alternative.
Limited Resources
- Finite Fuel Supply: While uranium is more abundant than fossil fuels, it is still finite, and extracting it in a sustainable way remains a challenge. Research into alternatives, such as thorium reactors, is ongoing but not yet widely implemented.
- Research and Development Needs: Innovation is crucial for addressing nuclear technology challenges, but it requires significant investment and a highly skilled workforce, both of which can be limited in supply.
Addressing These Challenges
While these challenges are complex, they can be addressed through:
- Innovative Research: Development of safer reactor designs (like Small Modular Reactors), alternative fuels (such as thorium), and advanced waste processing techniques.
- International Collaboration: Agreements like the Nuclear Non-Proliferation Treaty (NPT) and international oversight bodies (e.g., the IAEA) help regulate nuclear technology use globally.
- Policy and Education: Effective regulations, public engagement, and education can help demystify nuclear technology and build public trust.
- By confronting these issues, nuclear technology can contribute responsibly to sustainable development and energy needs.
Way Forward
- The way forward for nuclear technology lies in advancing safety, efficiency, and sustainability. Research into next-generation reactors, such as Small Modular Reactors (SMRs), molten salt reactors, and thorium reactors, promises cleaner, safer, and more efficient energy.
- The focus should be on reducing waste, improving fuel cycles, and enhancing reactor designs to prevent accidents. Additionally, fusion energy holds immense potential for a clean, virtually limitless power source, though technological breakthroughs are still required. Expanding nuclear energy as part of a diversified energy mix will be crucial for combating climate change, ensuring energy security, and reducing dependence on fossil fuels.
Conclusion
Nuclear technology offers significant potential for clean, high-efficiency energy production, with applications in power generation, medicine, and research. However, challenges such as safety concerns, waste disposal, and public perception remain. Continued innovation and strict regulation are crucial to realizing its full potential responsibly and sustainably.
GS - 3